The working conditions of wind turbines are largely determined by turbulent wind properties, as at typical wind speeds of about 10 m/s, turbulence inevitably occurs due to viscous interaction with the ground or water surface. Since the force evolutions on the rotor blade occur in fractions of a second, it is essential to understand the turbulent wind structures at these scales, which corresponds to a temporal resolution of about 100 ms and a spatial resolution of about 1 m. In different investigations it was shown that even very large wind turbines do not average out these short-term wind fluctuations, but that these fluctuations are even reflected in the fluctuations of the power output, which have completed the whole transformation process from the wind structures to the forces on the rotor blades and the transmission into the generator [1]. In the international guidelines for wind energy systems [2], the wind fluctuations on these scales are implicitly assumed to be Gaussian distributed, which is known to contradict the results from wind measurements. Instead, the operating conditions for multi-megawatt wind turbines with rotor diameters larger than 100 m correspond to non-Gaussian intermittent conditions, which are also often referred to as small-scale turbulence. Very large wind turbines as megastructures additionally operate at altitudes well above 100 m, where under certain meteorological conditions the turbulent and near-surface Prandtl layer transitions into the Ekman layer.
The working hypothesis of this subproject is that, first, offshore megastructures are exposed to wind field conditions that include both the turbulent properties of the Prandtl layer and the transition to the rather laminar Ekman layer, and that, second, the transition between the turbulent Prandtl layer and the Ekman layer has at least approximately universal structures, so that this flow phenomenon can be reproduced experimentally in a wind tunnel with a specially designed active grid (Fig. 1). From measurements and simulations of the meteorological conditions as well as from the wind tunnel experiments, a suitable basis is obtained to build stochastic wind field models that numerically reproduce the mentioned properties.

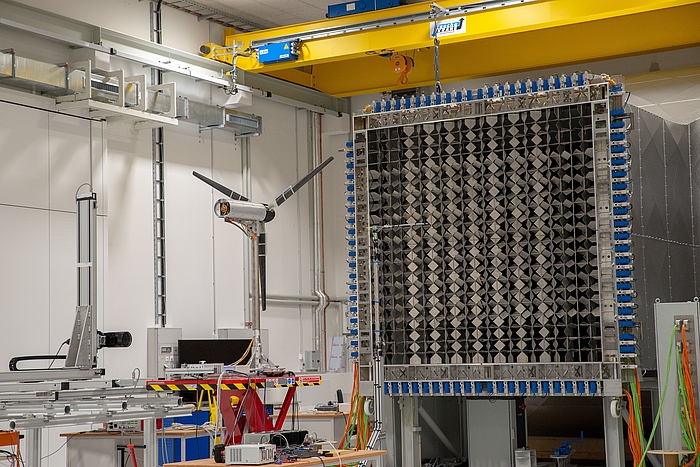
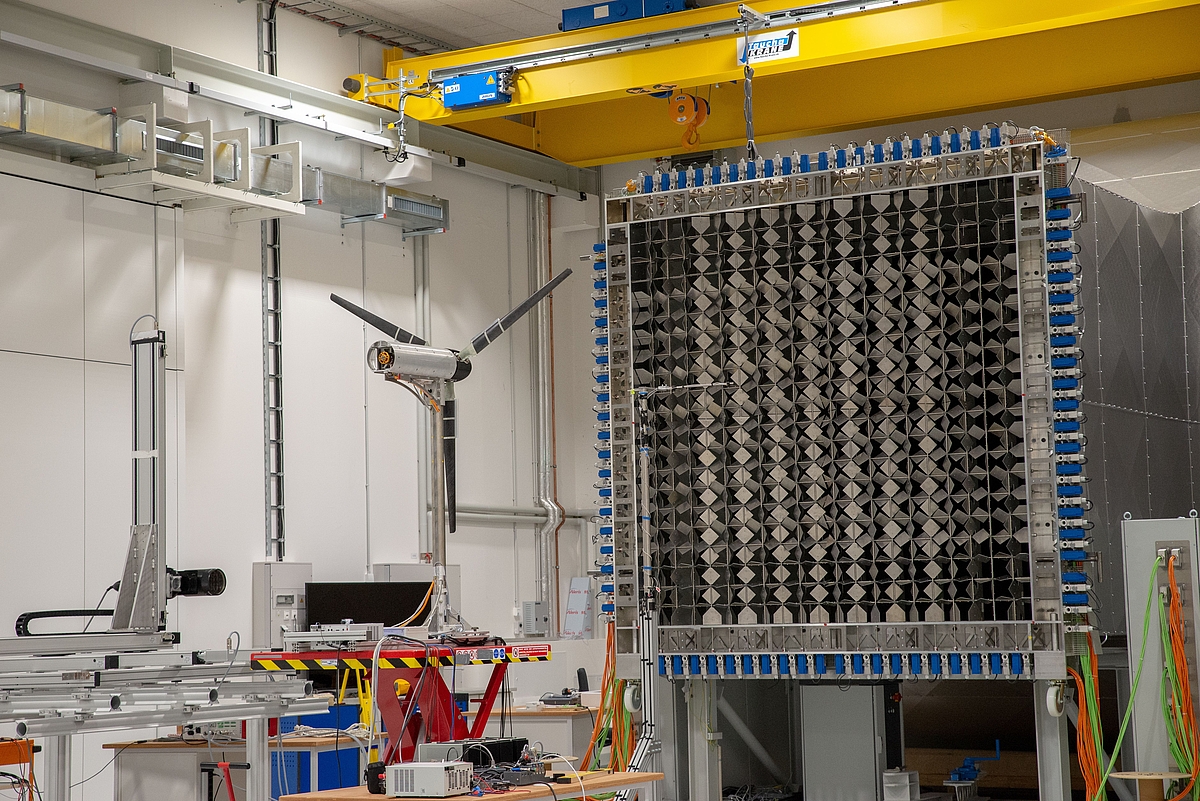
The main goal of this subproject is to create effective stochastic wind field models of this boundary layer as close to reality as possible, which will help to create a reduced, real-time capable overall model to a digital twin of an offshore wind turbine. To this end, a detailed characterization of the boundary between turbulent and non-turbulent flow regions must first be explored and reproduced in the wind tunnel under laboratory conditions. High-resolution measurements should enable the development of stochastic models, which are the subject of the second part of the subproject. The modeling of the boundary between turbulent and non-turbulent flow regions is essential for the development of the wind field model of the boundary layer. In the Collaborative Research Center, the subproject is placed in the cluster of wind and waves. The investigated wind fields are closely linked to the subprojects of the rotor (SP A02, B02, B03) and the control and monitoring and will finally be effectively integrated into the digital twin.
[1] Milan, P.; Wächter, M.; Peinke, J.: Turbulent Character of Wind Energy. Phys Rev Lett 110, 138701, 1-5, 2013.
[2] International Electrical Commission (IEC): Wind energy generation systems – Part 1: Design requirements, IEC 61400-1:2019 Ed.4, 2019.
Publications
-
2024: The fractal turbulent-non-turbulent interface in the atmosphere
Neuhaus, L.; Wächter, M.; Peinke, J. (2024): The fractal turbulent-non-turbulent interface in the atmosphere, Wind Energy Science, 9, 439-452
-
Juli 2023: Applying a random time mapping to Mann-modeled turbulence for the generation of intermittent wind fields
Yassin, L., Helms, A., Moreno, D., Kassem, H., Höning, L., Lukassem, L.J. (2023)
Applying a random time mapping to Mann-modeled turbulence for the generation of intermittent wind fields; Wind Energy Science, 8(7), 1133-11552 -
2022: Superstatistical wind fields from pointwise atmospheric turbulence measurements
J. Friedrich, D. Moreno, M. Sinhuber, M. Wächter, and J. Peinke (2022)
September 2022: Superstatistical wind fields from pointwise atmospheric turbulence measurements ; PRX Energy 1, 023006 -
2022: Generation of turbulence by means of active grids for wind turbine investigations
Neuhaus, L. (2022)
Generation of turbulence by means of active grids for wind turbine investigations, Doctoral dissertation, University Oldenburg -
2022: Numerical simulation of ice accretion on coated wind turbine blades
Yassin, K. (2022)
Numerical simulation of ice accretion on coated wind turbine blades; Doctoral dissertation, University Oldenburg -
Mai 2021: Exploring the capabilities of active grids
Neuhaus, L., Berger, F., Peinke, J., Hölling, M. (2021)
Exploring the capabilities of active grids. Experiments in Fluids 62(6), 130https://link.springer.com/article/10.1007/s00348-021-03224-5
Subproject Management
Institut für Physik & ForWind
Küpkersweg 70
26111 Oldenburg
Institut für Physik & ForWind
Küpkersweg 70
26111 Oldenburg
Institut für Physik & ForWind
Küpkersweg 70
26129 Oldenburg
Institut für Physik & ForWind
Küpkersweg 70
26129 Oldenburg